Part two of my series on air conditioning can be found here.
Air conditioning was initially a symbol of comfort and wealth, enjoyed by the wealthy in theaters and upscale homes. Over time, as technology advanced and costs decreased, air conditioning became more accessible to the general public.
With global warming, though, air conditioning has moved from being a luxury to being necessary for survival in many places. If you live in Phoenix or Houston and your air conditioner fails, staying in your house may be impossible and you may need to evacuate.
Air-conditioning now plays a central role in protecting public health in homes, workplaces, and public spaces. But, of course, not everyone can afford it. This is one of the biggest equity issues in the climate debate, with some saying, “we’ll rely on air conditioning” to address climate change. This essentially abandons the poorest in our society, as well as the animal world, to a hellishly hot world they did not create.
Given the enormous importance of air conditioning, I thought it would be useful to put together a few posts about it. This is part one: some background on the physics of air conditioning.
Heat engines
In thermodynamics, a heat engine is a device that converts thermal energy into mechanical work by exploiting the temperature difference between a hot and a cold reservoir.
A coal-fired power plant is an example of a heat engine: It takes heat from a coal-burning furnace, the hot reservoir, converts some of it to work, e.g., driving a generator to produce electricity, and rejects the remainder of the heat into the cold reservoir, which is the environment.
Note that you cannot convert heat to work with 100% efficiency. This is a consequence of the second law of thermodynamics (see appendix). The second law in fact allows us to derive exactly how much of the energy extracted from the hot reservoir can be converted into work:
where Th is the temperature of the hot reservoir, e.g., the furnace, and Tc is the temperature of the cold reservoir, usually the environment. Plugging in typical values of 80F and 600F for a coal-fired power plant1, you get an efficiency of around 50%.
This is the best you can do and, in the real world, you can’t achieve this: Very efficient coal-fired power plants tend to be around 40% efficient. This means that, for every 100 Joules of energy from burning coal, you get 40 Joules of electricity. The other 60 Joules are waste heat ejected into the environment. This necessary production of waste heat is a main reason that thermal power plants are usually sited next to rivers or lakes, which are used as heat sinks.
Air conditioners
What does this have to do with air conditioning? Well, an air conditioner is just a heat engine run backwards: you put work into it and it takes energy from the cold reservoir, the inside of your house, and ejects the energy into the hot reservoir, the outside of your house.
The efficiency of an air conditioner is usually expressed in terms of its Coefficient of Performance (COP): the ratio of the heat removed from the inside of your house (Qc) to the energy input required to remove that heat (W):
where Tc and Th are the temperatures inside and outside of the home and ∆T = Th minus Tc, the difference between the inside and outside temperature. You can think of the COP as the efficiency of your air conditioner
The important result here is that the efficiency of your air conditioner decreases as ∆T increases — e.g., as the outside temperature goes up.
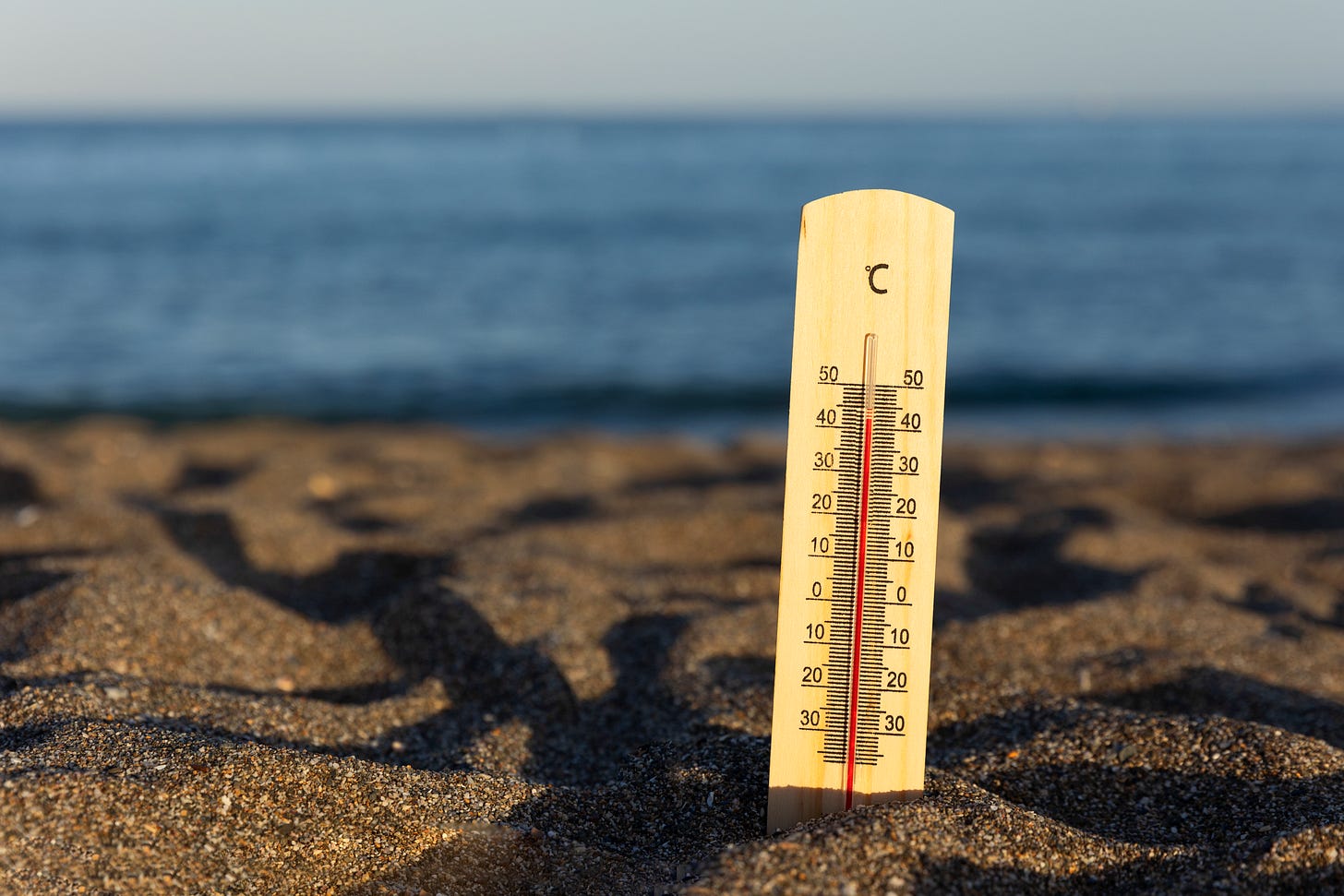
Rearranging the equation above, the energy W required to remove heat Qc from your house is proportional to:
Some numbers will illustrate the importance of this. If your house is at 75F and the outside temperature increases from 96F to 100F, then your air conditioner needs to consume 20% more energy to remove 1 Joule of energy from the inside of your house.
To maintain a constant temperature in your house, your air conditioner must continuously remove the same amount of energy that is entering your house. In other words, the cooling (Qc) of your air conditioner needs to match the energy flowing into your house.
If we assume that energy flowing into the house from the hot outdoors is set by Newtonian cooling, then Qc is also proportional to ∆T, so our expression reduces to:
Thus, the work required to keep your house at a fixed temperature Tc increases with the square of the temperature difference between inside and outside temperature, ΔT².
The impact on required energy
In this context, W is a measure of the amount of electricity you need to buy in order to air condition your house. As the temperature outside goes up, the energy required (and the amount of money you have to spend on it) increases as ∆T2.
Let’s use the same numbers from the previous example: you want to keep your house at 75F. If climate change has increased the outside temperature from 96F to 100F, the energy your air conditioner consumes increases by (100-75)2/(96-75)2 = 252/212 — this is an increase in energy consumption of 42%!
Averaged over an entire day, the increase will be less than this because ∆T is smaller for much of the day (e.g., at night) But the result is robust: climate change is driving exponentially increasing energy demand for cooling.
Can we air condition our way out of climate change?
Air conditioning is expensive and, because of climate change, it’s getting a lot more expensive. People with financial means, who work in air-conditioned offices and live in climate-controlled homes, can handle rising temperatures by simply paying for more electricity.
However, a significant portion of the global population lives the hot life. These people live in homes without air conditioning, work outdoors or in warehouses or kitchens with no climate control.
And even when people have access to air conditioning, they can struggle to afford it. This is well described by Jeff Goodell in his great Rolling Stone article about extreme heat in Phoenix:
This is the reality of air conditioning: great if you can afford it, terrible if you can’t. Any actual adaptation plan that relies on air conditioning will require massive government expenditures to air condition places that have historically not been air conditioned, like Chicago or Seattle. And there’s nothing the “we’ll adapt” crowd is less interested in than paying to help people adapt.
Appendix for the physics nerds: what determines maximum efficiency of a heat engine?
Why do coal-fired powerplant have a maximum efficiency of 50%? This maximum efficiency is determined by the second law of thermodynamics, which says that the entropy of an isolated system cannot decrease.
When a heat engine removes energy Qh from a heat reservoir at temperature Th, the entropy of the reservoir decreases by Qh/Th. Because of the second law, entropy must increase elsewhere to balance this decrease. This requires the engine to reject a quantity of heat Qc into a cool reservoir at a lower temperature Tc, which increases entropy of that reservoir by Qc/Tc.
For the engine to operate cyclically and not violate the second law, the increase in entropy in the cool reservoir must at least equal the decrease in the hot reservoir. This means Qh/Th = Qc/Tc for the most efficient engine possible.
By conservation of energy — the first law of thermodynamics — the work output of the engine W is the difference between the heat absorbed from the hot reservoir and the heat rejected into the cool reservoir: W = Qh - Qc.
Writing the efficiency of a heat engine as the ratio of the work output to the heat input, W/Qh, and substituting W = Qh - Qc, we can rewrite the efficiency as (Qh - Qc)/Qh, which reduces to 1-Qc/Qh.
And given that Qh/Th = Qc/Tc for a maximally efficient engine, this can be rewritten as 1-Tc/Th, which is the equation for efficiency presented at the beginning of this post.
What this shows is that the 40% efficiency typical of thermal power plants is not lazy engineering. Rather, this is required to not violate the second law of thermodynamics.
Update: Let me emphasize that this is an idealized calculation and the exact increase in energy demand with temperature will depend on a lot of factors I have not included (just like the physicist’s friend, the spherical cow spraying milk in all directions). For example, the assumption of Newtonian cooling is only true if no power is dissipated inside the house. If you’re running a bitcoin farm or marijuana grow operation in your house, then Newtonian cooling is not a great assumption. It may be that realistic systems are closer to linear in ∆T, which would imply an increase of energy demand of 20% for the temperature increase of outside temperature of 96F to 100F. Thus, the essential conclusion — that energy demand for cooling rises rapidly with outside temperature — is robust.
Related material
Spare a moment for those living “the hot life”
Adaptation to climate change will be an absolute nightmare
you have to convert these temperatures to Kelvin (300K and 589K) before you plug them into the equation.
AC is quickly becoming a survival necessity. I would go so far in saying that, at some point, it will be declared a fundamental human right that can't be denied, especially to those living in certain regions affected by extreme heat waves. Therefore, I don't believe it will help to ask people to give up AC, no more and no less than it would be to ask them to give up heating. Telling them to be parsimonious may help to postpone a bit the problem, but won't solve it. Whereas, we should face reality and accept that AC also will steadily pile up on the top of all the electric energy consumption. This is only a reason more to go all in with renewables, impose on new buildings efficient home insulation, heat pumps, green roofs, solar panels, energy storage systems, thermostats, ceiling fans, educate people how to optimize their homes’ thermodynamics, and for those who can’t afford it, create public facilities with air conditioning as “heat shelters,” etc.
So, can we air condition our way out of climate change? Of course not. Climate change will stay with us for generations to come and will have to be fixed on a completely different level. But I think it is time to stop preaching to people about “AC chastity,” telling them to “drink more water.” We must develop a serious and rational long-term plan that integrates it into the energy grid, without collapsing it. The first thing that must change are the simplistic and sterile narratives that surround this topic. Time is running out!
Another problem I didn't see mentioned here. Right now air conditioners rely on Hydrofluorocarbons (HFCs). Which are a greenhouse gases that stay in the atmosphere for 15 years, and are 1,000-9,000 times more potent than CO2.